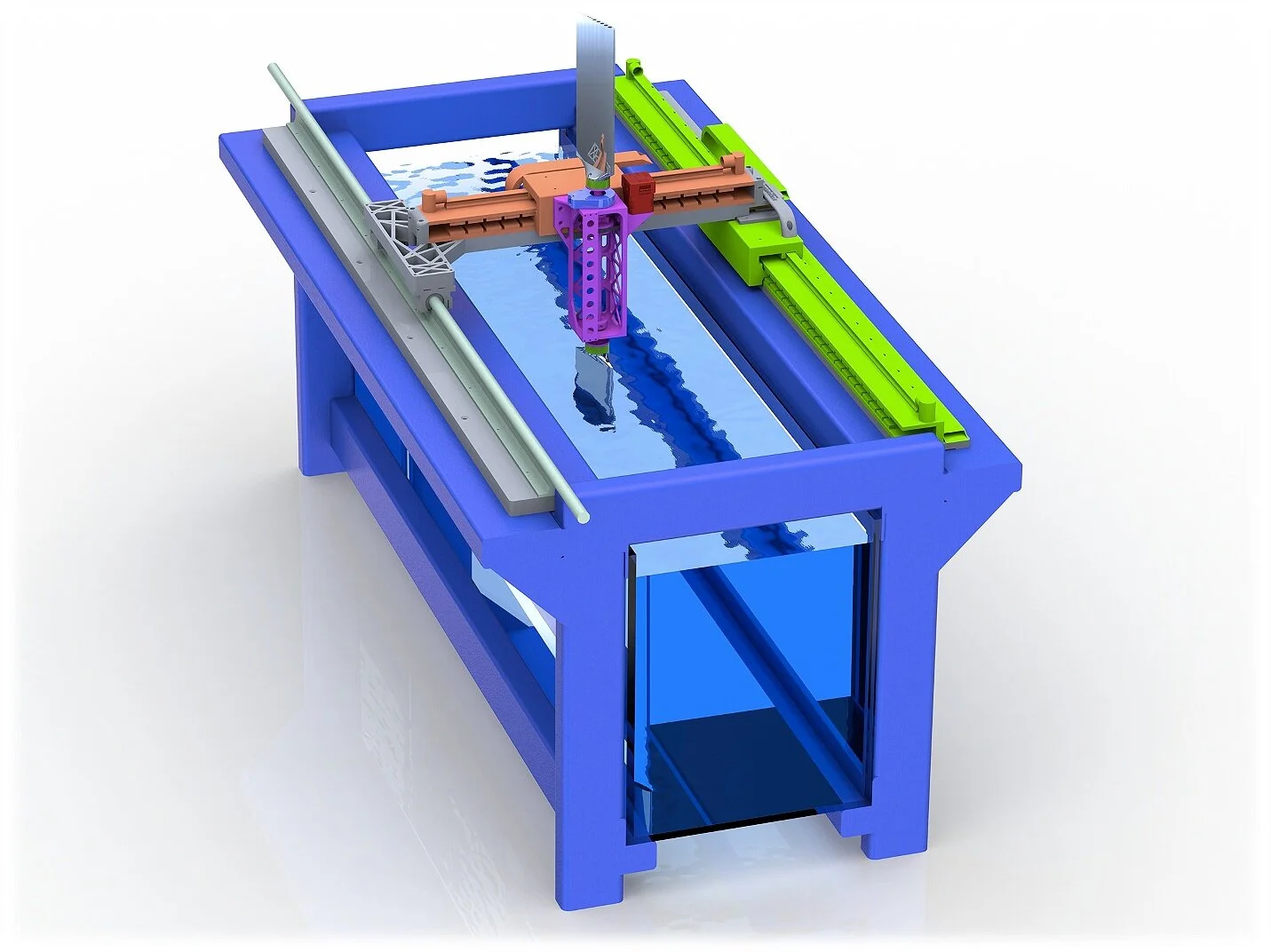
Efficient Flapping Airfoil Propulsion
Cyber-Physical Fluid Dynamics
I utilize the Cyber-Physical Fluid Dynamics (CPFD) Facility to conduct my research on efficient oscillating airfoils. This facility combines a computer controlled XY towing tank and flowing water channel with a robust force-feedback system. CPFD is a powerful technique that unifies the flexibility of imposing arbitrary parameters of computational fluids with the real-time speed and infinite resolution of experimental fluids. An oscillating airfoil is submerged in the fluid in the 26’ flowing water tank, and the resulting fluid forces and moments of the experiment are measured in real-time with a 6 degree-of-freedom force/torque sensor and sent to the computer control system. These force readings are then coupled with any virtual forces or kinematics that the user imposes. From this, a velocity command is calculated to satisfy Newton’s second law and outputted back to the oscillating airfoil on the experimental side. Repeating this cycle creates a system where the flapping wing moves in response to both the fluid forces acting on it as well as any additional forces and kinematics we specify virtually, effectively coupling experimental and computational methods. The computer control portion of CPFD will allow parameters such as mass to be arbitrarily specified while still measuring the unsteady and complex physical fluid forces that occur during oscillation, overcoming limitations of traditional experimental flapping wing propulsion research. The facility is also equipped with a laser doppler velocimeter (LDV) to measure the instantaneous water channel speed and an Nd:YAG laser to perform particle imaging velocimetry (PIV) to study the underlying vortex dynamics of the unsteady aerodynamics.
Self-Propulsion of an Oscillating Airfoil
My latest research investigates the performance of a self-propelled heaving and pitching airfoil. Self-propulsion is achieved when the airfoil is freely allowed to accelerate and decelerate in response to the instantaneous forces it experiences until it reaches a steady-state cruising velocity. This approach differs from traditional experimental flapping wing propulsion in which the airfoil is generally tethered and an arbitrary incoming flow velocity is implemented (usually to satisfy some Reynolds number criteria). Self-propulsion more accurately models real-world conditions in which an object will continue to accelerate or decelerate until the thrust generated equals to the drag. Because self-propulsion precisely couples the airfoil’s cruising velocity to the forces it generates, this research has the exciting potential to guide design of self-propelled flapping wing vehicles such as micro-air vehicles (MAVs) and autonomous underwater vehicles (AUVs).
To see how self-propulsion is implemented in the CPFD facility, please watch the video below:
Addition of Passive Dynamics to an Actively Heaving Airfoil to Improve Performance
In this project, the heaving motion was prescribed and the pitching motion was generated passively from fluid forces and virtual torsion springs implemented with CPFD. Because the torsion springs were virtually implemented, the spring constants and pivot location could be arbitrarily changed, allowing for a parameter space that would’ve been prohibitively large with standard experimental techniques. With the appropriately tuned spring constant, the torsion spring could produce up to an order of magnitude increase in thrust compared to a purely heaving airfoil. This increase comes at no cost to efficiency and requires no second actuator. This is important as it decreases cost, complexity, and weight in the design of MAVs and AUVs, which generally have constrained mass and energy budgets. The pivot location could also be modified to force the airfoil into thrust conditions in which a reverse von Kármán wake is formed to create a time-averaged jet (shown to below on the left) or into drag conditions in which complex shedding results in recirculating regions (shown below on the right).
Effect of hybrid-heave motions on the propulsive performance of an oscillating airfoil
In this project, a sports-mimetic approach was taken to study the unsteady airfoil motions inspired by sailing aerodynamics. Sailors can generate extra propulsion by using bodyweight motions to roll the boat along its longitudinal axis, creating a “sail flicking” motion. Because sailors do not travel directly into the wind, the boat travels at an angle to the relative wind it experiences. The “flicking” sail will therefore oscillate at non-perpendicular angles to the incoming flow, in a motion we call “hybrid-heave.” Studying these hybrid-heave motions with a NACA 0012 airfoil, a distinct “high lift” mode (shown below to the left) and “low lift” mode (shown below to the right) were discovered. The high-lift mode delivers significantly increases the lift compared to that of a static airfoil, whereas the low-lift mode can generate less lift than a static airfoil. To read our published work on this topic, click the button below to be directed to the Journal of Fluids and Structures article.